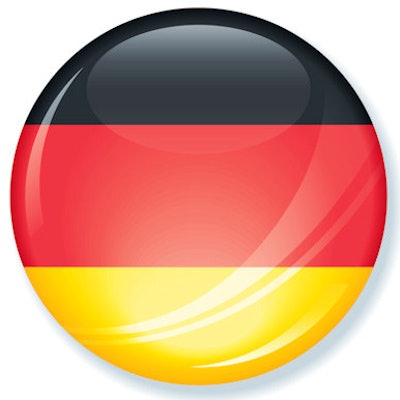
Measuring the energy of gamma rays emitted during helium-ion radiotherapy could provide real-time verification of the beam's range in the patient. Researchers in Germany and Portugal have demonstrated a spectroscopy unit that can spot the signatures of 19 distinct nuclear transitions that occur when helium ions interact with various atomic nuclei near the end of the beam path. The technique will help define the size of the range error in particle therapy, allowing more precise treatments with greater sparing of healthy tissue.
The advantages of particle therapy over x-ray radiotherapy are well established. Whereas photons deposit energy along their entire trajectory, irradiating large volumes before and behind the target, the dose delivered by protons and heavier ions is concentrated at the Bragg peak, just before the particles are brought to a halt by interactions with the medium.
This property of charged particles arises because their interaction cross-section increases as they slow down, meaning their range can be tuned by adjusting their initial velocity. Even with precisely controlled beam energy, however, errors from other sources -- patient imaging and setup, for example -- make the actual range in clinical applications uncertain.
It is necessary, therefore, to measure the beam range in vivo, so that physicists can confirm that the particles' energy has been delivered to the correct depth in the patient. One method involves the use of PET to image the unstable isotopes created when the beam interacts with tissue. The problem with this technique is that, typically, several minutes elapse before the isotopes decay, during which time biological washout can compromise the signal.
A more immediate indication can be achieved by measuring the secondary charged particles that are also produced by the beam, but as these are scattered by atomic nuclei on their way to the detector, the picture that emerges has poor spatial resolution.
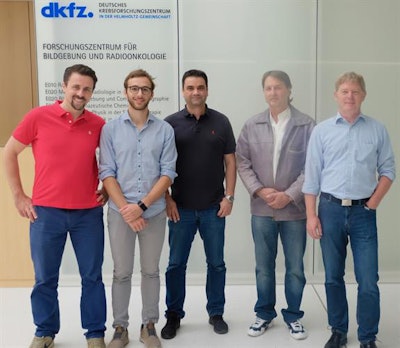
Prompt gamma spectroscopy (PGS), the approach taken by Riccardo Dal Bello, at Heidelberg University and the German Cancer Research Center (DKFZ); Paulo Martins, PhD, at DKFZ and the University of Lisbon; and a team from DKFZ, Heidelberg University, and the Max Planck Institute for Nuclear Physics in Germany, achieves the near-instant response of secondary charged-particle tracking while matching the spatial resolution of PET.
Dal Bello and colleagues fired beams of helium-4 (He-4), at energies similar to those used clinically, into acrylic or water targets. At 90° to the beam, at the point where they calculated the Bragg peak would be, the researchers placed a gamma spectroscope consisting of two concentric detectors (Medical Physics, 14 May 2019).
The primary inner detector made high-resolution measurements of the energy of photons exiting the target. The outer detector was used for anticoincidence detection, meaning that it spotted photons that had been scattered inelastically from atomic nuclei in the inner detector. In such cases, the energy recorded did not reflect the true energy of the photons that left the target, so these measurements were discarded.
Dal Bello and colleagues found that the measured spectra corresponded to specific, identifiable nuclear interactions.
"The first interaction is always the collision between [He-4] and [oxygen-16 (O-16)]," said Joao Seco, PhD, of DKFZ and Heidelberg University, who led the team. "Such collisions can produce a large number of nuclei at different excited levels. Therefore, from a single collision between [He-4] and [O-16], multiple independent variables can be extracted, which can be used to estimate the energy of the collision."
Observing the excitation states of the nuclei produced in collisions, then, gives a snapshot of the beam energy at that point on its trajectory. And because of the relationship between the energy of a particle and its range, knowing the energy at that point provides a way to determine how far the particles still have to go and where the dose will be concentrated.
"The next step is the direct measurement of all the energy-dependent cross-sections for the prompt gamma production with proton, helium, and carbon beams at clinically relevant energies," Seco noted. "At the moment, safety margins are added to the irradiated tumor volume to account for the range uncertainties in proton or carbon ion centers, so the first use of PGS would be to study range errors, reduce safety margins for a variety of treatment sites, and produce quantitative data."
Marric Stephens is a freelance science writer based in Bristol, U.K.
© IOP Publishing Limited. Republished with permission from Physics World, a website that helps scientists working in academic and industrial research stay up to date with the latest breakthroughs in physics and interdisciplinary science.