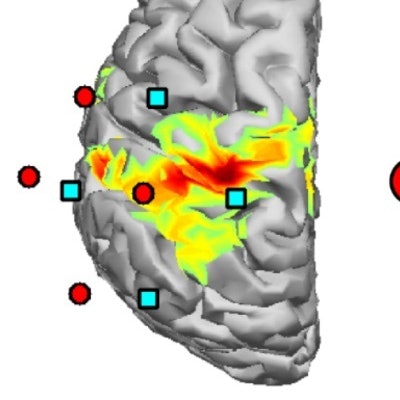
Researchers from Advanced Telecommunications Research Institute International (ATR) and Ricoh in Japan have used a multidirectional functional near-infrared spectroscopy (fNIRS) system for human neuroimaging.
Instead of increasing probe density on the scalp, which is impractical, the team increased the number of optical paths that the NIR light could take from source to detector, using a single probe. This approach produces a richer dataset and could improve 3D visualization of brain activity.
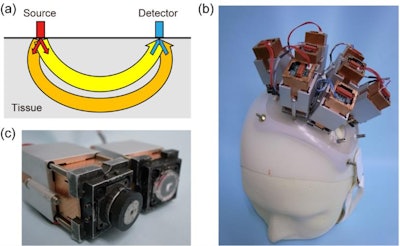
By transmitting NIR light to the scalp and detecting the reflected light, fNIRS can measure changes in concentration of deoxy- and oxyhemoglobin, based on their different specific absorption spectra. These changes arise from neuronal activation in the cerebral cortex and, similar to functional MRI, give an indirect measure of neural activity.
The benefits of fNIRS include its portability, low cost, and adaptability to different populations -- for example, babies and elderly patients -- compared with large-scale imaging techniques such as MRI.
Diffuse optical tomography
One obstacle when employing fNIRS is the scattering produced when NIR light is transmitted into biological tissue, which makes image reconstruction difficult. The number of activation sources in the brain outnumbers the number of detectors on the scalp, and estimating where the source locations are is a difficult task. Recently, the use of diffuse optical tomography (DOT) has allowed 3D visualization. However, DOT requires more detailed measurements, for example, using detectors spaced just 13 mm apart.
The DOT algorithm first constructs a forward model using an anatomical model of the head, figuring out how the NIR light has traveled. Inverting this solution and combining it with measured data allows 3D visualization of useful brain images.
Practically, increasing probe density is a problem; it takes longer to optimize scalp contact and each probe's position must be noted with high accuracy -- this leads to greater patient burden.
Takeaki Shimokawa, Toshihiro Ishii, and their research groups developed a multidirectional fNIRS system that can increase the measurement data, without increasing the number of probes. Instead of a single optical path from source to detector, the device produces multiple paths by changing the emission and detection angles (Biomedical Optics Express, 1 March 2019, Vol. 10:3, pp. 1393-1404).
The system has four sources and four detectors, with four different angles of emission and of detection, and it produced 16 times more data than monodirectional fNIRS. The researchers tested the device on a single subject who performed a grip-task to evoke activity in sensorimotor brain areas. The subject performed the same task during an fMRI scan for comparison. The researchers noted that the system cannot currently deal with hair; thus, they chose a subject with no hair.
The team compared the observed fNIRS data with simulated fNIRS data based on the fMRI image. The observed data correlated well the simulated data.
The fNIRS data are dependent upon the source-detector pair, the source direction, and the detector direction. Because fNIRS data mainly depend on source-detector pair positions, the team subtracted this pair factor from the fNIRS signal and extracted the directional-dependent signals. They then compared these with the simulated fMRI data. The correlation here was lower, which is to be expected as the directional signal is about five times weaker than the positional signal.
The authors noted that this is the first detection of direction-dependent fNIRS signals based on brain hemodynamics.
Visual inspection showed good overlap between the hemodynamic response functions measured using the fNIRS probes and the fMRI maps, evidence that the system works.
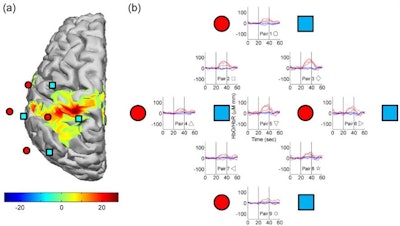
The researchers reconstructed DOT images and compared these to the fMRI image. They quantified the comparison by looking at the errors in localization, computed as center of mass distances between oxyhemoglobin (fNIRS) and fMRI maps. The error values were within the expected range.
Future directions
The team hopes to test the system on more subjects, as well as further optimize the multidirectional measurement by reducing assembly errors in source probes and better modeling of the NIR light sources. Finally, the researchers plan to miniaturize the probes for practical use, as well as for measurements performed on subjects with hair.
Michael Asghar is a doctoral student contributor to Physics World. He is studying neuroscience at the University of Nottingham in the U.K.
© IOP Publishing Limited. Republished with permission from Physics World, a website that helps scientists working in academic and industrial research stay up to date with the latest breakthroughs in physics and interdisciplinary science.