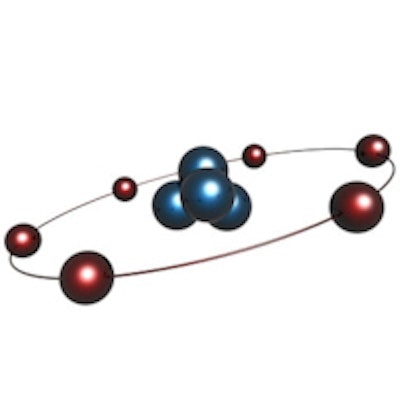
Delegates at the 52nd annual meeting of the Particle Therapy Co-Operative Group (PTCOG 52) heard how introducing imaging techniques into the proton therapy workflow can improve treatment accuracy.
The finite range of a proton beam means that even small geometric and anatomic variations can lead to dose discrepancies when delivering proton therapy. Speaking at last week's PTCOG 52 meeting in Essen, Germany, Martijn Engelsman, PhD, described how proton therapy can benefit from various image-guidance techniques.
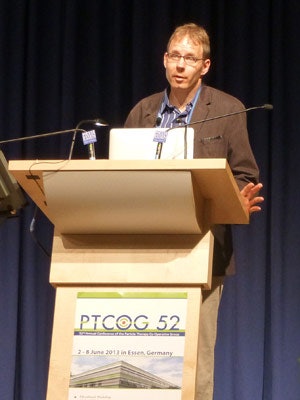
"The machine is not the problem," said Engelsman, from Delft University of Technology in the Netherlands. "The problem is the patient." He explained that while modern treatment systems can deliver protons with an accuracy of less than 0.5 mm, range uncertainties arising from setup errors, CT number-to-proton stopping power conversions, intrafraction motion, and interfraction anatomy variation all can degrade the proton dose distribution.
Engelsman began by discussing some basic range uncertainties. Currently, the total background error, due to uncertainties in CT imaging, conversion of CT data to proton stopping power, and dose calculation, is estimated to be in the range of 3% to 3.5% plus 1-3 mm. "Improvements in image guidance can probably reduce this by a factor of two," he explained.
To realize an accuracy gain of around ±3 mm, one option is to use dual-energy CT for dose calculations. This method is more robust to uncertainties in tissue composition than standard CT, as it incorporates elemental composition information when calculating proton stopping power ratios. Engelsman noted, however, that the accuracy of this technique has not been fully validated under clinical circumstances such as beam hardening, scatter, and noise.
Another possibility would be to implement proton radiography or proton CT, which offer direct measurement of proton stopping power. On the downside, these methods suffer from limited spatial resolution and long reconstruction times; they are also still in the development phase.
Engelsman described some medium- and long-term goals. He noted that his five-year goals aim to significantly improve accuracy but, more importantly, help us understand how best to use advances in imaging and help define the right questions. The 10-year goals, meanwhile, represent "the sky is the limit" aims.
In five years' time, he said, we should be using 5-mm resolution proton CT or radiography as a one-time, per-patient rough evaluation of CT conversion. In 10 years, we should aim for low-dose, daily isocentric proton CT for dose recalculation. Another option could be to implement one-time, high-resolution proton CT, which is then deformably registered onto a daily imaging set.
In vivo dosimetry
The next stage in improving treatment accuracy lies in the use of in vivo proton dosimetry. Visualization of the beam during delivery would provide real-time validation of machine behavior and proton stopping power conversion, and indicate any significant anatomy changes.
Engelsman described the two main technologies being lined up for in vivo dosimetry. The first is the use of PET imaging to visualize positron emitters generated as the therapeutic beam travels through the patient. This method is already being applied clinically, both online and offline, and can deliver an accuracy of 1-2 mm in favorable locations. It does, however, suffer from biological washout of the signal and low counting statistics.
The second option under investigation is prompt gamma imaging, which is performed online and provides immediate data. While this method is particularly suitable for determining beam range, it is still in the early stages of development, Engelsman noted. "There are plans to bring a prompt gamma prototype into the clinic in the next one or two years," he said.
In five years' time, Engelsman said, we should aim for routine use of limited accuracy (10 mm and above, for example) in vivo dose monitoring, to complement offline plan and field quality assurance (QA). The 10-year goal, he suggested, is millimeter-accuracy real-time dose reconstruction. This could provide a basis for implementing dose-guided proton therapy, along with in vivo QA of the adapted treatment plan.
Anatomy variations
Finally, Engelsman explained how imaging can address interfraction anatomy variations arising from patient weight changes, for example, or tumor shrinkage. Options include performing cone-beam CT in the treatment position or an out-of-room diagnostic CT, which offers higher contrast and image quality and can be used for dose recalculation.
The goal for today Engelsman said, is to ensure a rapid rollout of cone-beam and diagnostic CT. In five years, we should have clinical experience in routine, full 3D/4D imaging, along with smooth image acquisition and image matching. In 10 years, he envisioned, we may perhaps see the integration of MR guidance into proton therapy.
Much more important than volumetric imaging, Engelsman continued, is how we will use these images, especially for replanning. Currently, replanning is an offline process that can take two to three days. In five years, we should achieve online evaluation of plan accuracy and daily selection of a plan of the day based on in-room CT.
In 10 years, Engelsman said, we should be aiming to achieve 10 sec automated online plan reoptimization, while the patient is on the treatment couch. He noted that this level of automation would effectively remove the physicist and the physician from the treatment preparation process, thus requiring a high level of faith in the treatment planning system. Furthermore, in vivo dosimetry then becomes a necessity as a replacement for off line dosimetric plan-specific QA.
Engelsman emphasized that the main priority at present is to be able to retrieve information regarding the quality of dose delivery -- even by simply introducing existing 3D imaging technology into the proton therapy workflow -- by means of rapid dose recalculation, and to replan where required. "The five-year goals are certainly within reach," he concluded. "They're certainly not the end result, but they will help us to formulate what we want to achieve in 10 years' time."
Related links
© IOP Publishing Limited. Republished with permission from medicalphysicsweb, a community website covering fundamental research and emerging technologies in medical imaging and radiation therapy.